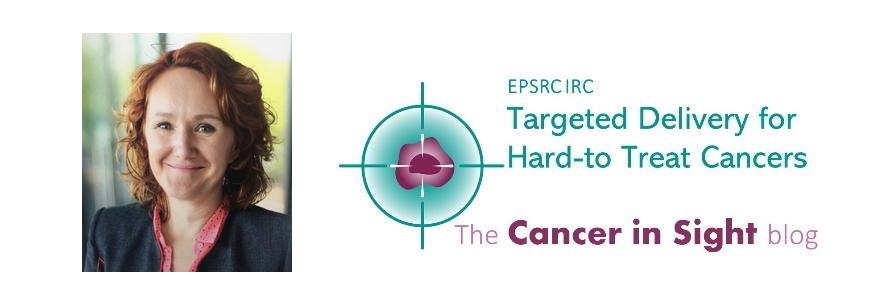
Dr Ljiljana Fruk, Associate Professor of Bionanotechnology at the University of Cambridge and IRC Investigator, considers the relationship between the fiction and fact of nanotechnology and its potential as part of the IRC to help target hard-to-treat cancers.
Not many research fields have been as hyped and plagued by misinformation as nanotechnology. From Michael Crichton’s infamous nanorobots in Prey to the latest James Bond DNA-attacking nanotech in No Time to Die, fictional stories about the nanotech doomsday abound.
Fact meets fiction
Besides tickling our imagination and providing a compelling image of yet unknown worlds, science fiction has been a faithful companion of scientific developments – and on many occasions a good predictor of the future to come. In 1966, when the Cold War was raging and spy movies were huge in Hollywood, one film considered the fight between good and evil from a different perspective. Fantastic Voyage by Richard Fleisher describes the crew of an atomic submarine shrunk to microscopic size and injected into the bloodstream of a dying patient to dissolve a blood clot on the brain. The science fiction author and Professor of Biochemistry, Isaac Asimov, was commissioned to write the screenplay alongside other writers to make the story more realistic. It is likely the writers were aware of the physicist Richard Feynman’s famous lecture to the annual American Physical Society in Caltech in 1959 which described worlds the size of atoms and the idea of a surgeon small enough to be swallowed to perform surgery.1
Clearly, we cannot shrink the navigator and drive the submarine into the tumour as the Fantastic Voyage crew did, but we can use both the intrinsic properties of the nanomaterial, their size and chemical structure, as well as additional modifications so they can reach target cells and spare the healthy ones. No longer limited to the narrative of science fiction, nanomedicine is playing a meaningful part in targeting hard-to-treat cancers. Dr Ljiljana Fruk Associate Professor of Bionanotechnology and IRC Investigator
Figure 1: Transmission electron micrograph of salmon DNA-gold nanoparticle nanoflower
These initial fictional, speculative ideas are slowly coming to life, although perhaps not in a form hoped for by science fiction fans. There are no nanorobots that can be injected into our body to deal with viruses and repair our tissue, and there are no secret alien messages encoded in intricate nanoflowers. That said, when I read Peter Hamilton’s 1995 science-fiction trilogy, The Nano Flower featuring a flower containing alien DNA2, I could not resist sending the author a transmission electron microscopy (TEM) image of my research team’s own nano flower made of gold nanoparticles and salmon DNA, which came to life as we were making DNA-based memory devices.3 Our nano flower was not made from alien DNA, but you can imagine how excited we were to see this beautiful nanostructure under the microscope.
Biological machines
Our DNA does not look like a nano flower, but we are full of intricate nanomachines floating through our body: protein assemblies that repair and rebuild our tissue with precision evolved over millions of years. Although powerful, our biological machines wear out and become damaged, leading to diseases, which, if left untreated, enter the vicious cycle of continuous damage and ultimate dissolution. As the sizes of these biological machines and other functional biomolecules range from sub to several tens of nanometres, it is not surprising that we are interested in harnessing the properties of nanomaterials to tune biomolecular activity, promote tissue repair and deal with disease.
Although the field of nanomedicine only truly came to life at the end of the 1990s, established with the publication of Robert Freitas’ academic textbook,4 there are indications that some forms of silver and copper colloids (suspensions of nanosized metal particles) were used as antibacterial compounds in ancient Egypt. Today, nanomedicine is a field that covers both the diagnostic and therapeutic aspect of disease; early diagnostics being enabled by nanomaterial – enhanced biosensors, and therapies benefiting from drug nanocarriers and intrinsically active nanomaterials such as antibacterial silver nanoparticles.
Coming of age
However, the field is not free of critique, and a number of articles published in the past few years question the efficiency of nanocarriers and the value of nanotherapeutics. While it is true that the field appears not to have delivered the promise of 30 years ago, the rational design of nanomaterials that really took off 10 years ago provides a new platform, which takes into account the biology of disease, more suitable validation models and a much deeper understanding of material-biological system interaction. Considering the timescale needed for a drug formulation to get from the lab into the clinic (often 10 or more years), the next decade is likely to see a significant increase in the number of nano formulated drugs on the market as nanomedicine realises its full potential.
Nanocarriers for hard-to-treat cancers
What makes the field exciting? First and most important is the need for new therapies, particularly in the field of oncology. Although we have come a long way in designing efficient therapeutic approaches to treat cancer – and are on the verge of turning certain types of cancer into chronic diseases – we still rely on protocols established decades ago. The cocktails of chemotherapeutics administered to patients have many side effects, and we now know that the healthy cells damaged during chemotherapy can lead to the development of other diseases and secondary cancers. Improving therapeutic strategies will require changes within health systems, such as putting more emphasis on prevention and early diagnostics, but we also urgently need improved therapeutic regimes. This requires clever design and better pre-clinical validation of drug formulations, more targeted treatments, less frequent administration and more combination treatments tailored to individual patients. Drug nanocarriers can play a significant role in providing this new generation of therapeutics and help us transition a new era of targeted and precision medicine.
Back to nature
The first nanomaterial-based formulation approved by drug agencies was a liposome loaded with chemotherapeutic doxorubicin in 1995.
Figure 2: Doxil is composed of liposomal shell protecting chemotherapeutic doxorubicin
Made of phospholipids similar to those that build our cell membrane, liposomes provide a biocompatible shell capable of solubilising usually hydrophobic (and therefore difficult to formulate) drugs. Many similar formulations based on lipids (lipid nanoparticles, micelles) followed soon after the approval of liposomes, meanwhile almost every type of nanomaterial has been explored for healthcare application. However, in order to come up with truly biologically effective nanomaterials, we need to reject the idea of an all-in-one solution and always try to go back to nature to find designs that are not only more sustainable but make more chemical and biological sense. It is unlikely that one type of nanocarrier will be able to deal with highly heterogenous solid tumours such as pancreatic cancer, which will more likely require two or three formulations, each dealing with a different aspect of the disease.
Changing the narrative
The IRC team are trying to explore these routes, focusing on biopolymer and metal-organic framework based nanocarriers to both deliver the chemotherapeutic and reprogramme the cancer microenvironment. Our approach is attempting to improve the efficacy of therapeutics for hard-to-treat cancers and involves collaborating with cancer biologists and clinicians during the design phase as well as chemical engineers in order to achieve the right balance between manufacturing, cost of design, and anti-tumour activity. Our design moves away from inorganic nanoparticles and takes into account mechanical and chemical properties of the nanocarrier coat, to enable diffusion into dense, solid tumours while providing favourable degradation pathways post-delivery.
When we talk about the design of nanocarriers, we often get asked about targeting. Clearly, we cannot shrink the navigator and drive the submarine into the tumour as the Fantastic Voyage crew did, but we can use both the intrinsic properties of the nanomaterial, their size and chemical structure, as well as additional modifications so they can reach target cells and spare the healthy ones. No longer limited to the narrative of science fiction, nanomedicine is playing a meaningful part in targeting hard-to-treat cancers.
References
1 Feynman, R. (1960). There’s plenty of room at the bottom. Engineering and Science 23(5),22-36.
2. Hamilton, P (1996). Nanoflower.
3. Yu-Chueh Hung, Wei-Ting Hsu, Ting-Yu Lin, Lj. Fruk, Photoinduced write- once read-many-times memory device based on DNA biopolymer composite, Appl. Phys. Lett. 2011, 253301.
4. Freitas Jr, R. (1999). Nanomedicine. CRC